So, What Is Quantum Computing?
Quantum computing, is a field that blends a variety of disciplines including quantum hardware and algorithms, is poised to revolutionize our ability to solve problems that are currently beyond the reach of even the most advanced supercomputers - highly advanced computing machines designed to perform complex calculations at incredibly high speeds, often used for tasks like climate modeling, molecular simulations, and other large-scale scientific computations.. Right now, these quantum computer units are big and bulky, so while this technology is still developing, its potential is immense.
At the core of quantum computing lies quantum mechanics, the study of particles at the atomic and subatomic levels, you can further come to terms with their bizzare and frankly, spooky behavior by understanding superposition and quantum entanglement here. This branch of science uncovers natural principles that are counterintuitive and often perplexing, yet they form the foundation for the behavior of everything in our universe. Quantum computers like quantum mechanics operate in a way that’s entirely different from classical machines.
Imagine a computing challenge so complex that it would take a traditional computer thousands of years to solve, but with the power of quantum computing, that same puzzle could be cracked in a matter of minutes. The reason lies in the unique way quantum computers process information, using quantum phenomena to calculate in a manner that is not just faster but fundamentally different.
As we continue to explore and develop quantum technology, we edge closer to a future where problems once thought insurmountable become manageable. Quantum computing isn't just an evolution; it's a leap into a new paradigm of problem-solving. I’ll try my best to help you understand what quantum computing is by breaking down the fundamental systems it relies on, what it’ll be used for and what its advantages are.
Firstly, let’s discuss the prime factors of Quantum Mechanics.
1. Superposition - At the center of quantum mechanics is superposition, where a quantum particle or system doesn’t just settle for one possibility. Instead, it represents a combination of multiple possibilities at once. This principle is key to the extraordinary potential of quantum computing.
2. Qauntum Entanglement - Another intriguing phenomenon is entanglement. When quantum particles become entangled, they form a connection that defies ordinary logic. These particles become so strongly correlated that the state of one instantaneously affects the state of the other, no matter the distance between them.
3. Interference and Decoherence - Entangled quantum states don’t just sit still—they interact. This interaction, known as interference, allows quantum states to combine in ways that amplify certain probabilities while diminishing others. It’s through interference that quantum computers can explore multiple outcomes simultaneously. But, quantum systems are still delicate. Over time, they can lose their quantum properties through another semi-opposing process called decoherence. When this happens, the system collapses into a single, definite state that aligns with the familiar laws of classical physics. Managing decoherence is one of the significant challenges in building stable quantum computers.
Bits vs Qubits
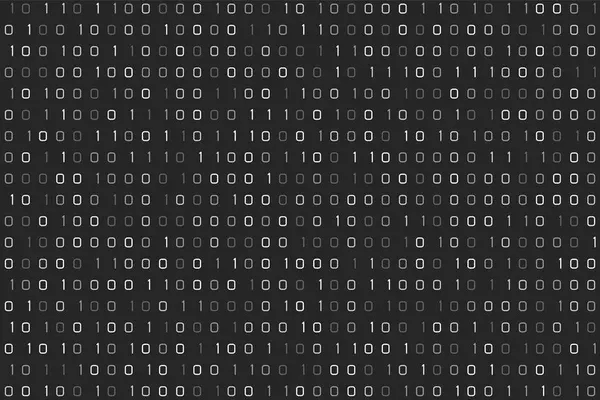
In classical computing, data is stored and processed using binary bits—zeros and ones. Each bit is like a tiny switch that can be either off (0) or on (1). Quantum computing, however, operates on a different principle entirely, using quantum bits, or qubits, which can hold far more information than a traditional bit.
Unlike a classical bit, which can only be a zero or a one, a qubit can exist in a state of superposition. This means a qubit can represent both zero and one simultaneously, as well as any weighted combination of these states. The power of qubits truly shines when they are combined. For example, while two classical bits can store only two distinct values, two qubits in superposition can encode four possibilities at once. This scaling continues exponentially: three qubits can store eight values, four qubits twelve, and so on.
There’s a catch though: despite this vast potential, each qubit can only output a single bit of information at the end of a computation. The magic of quantum algorithms depends on their ability to manipulate and store information in ways that classical computers simply cannot, potentially offering massive speedups for certain complex problems.
As classical computers, built on silicon chips and superconductors, continue to advance, we are rapidly approaching the physical limits of what these materials can achieve. Quantum computing could be the next leap forward, particularly for solving problems that are currently intractable with classical methods.
Major tech giants like Microsoft, IBM, Google, Amazon, and Waze along with innovative startups such as Rigetti and IonQ, are heavily investing in this groundbreaking technology. Experts predict that by 2035, quantum computing could grow into a USD 1.3 trillion industry, driving forward the next era of computing.
What Are Qubits And How Do They Actually Work?
Qubits are the fundamental units of quantum computing, created by manipulating and measuring quantum particles—the smallest building blocks of our universe. These particles can include photons, electrons, trapped ions, and atoms. In some cases, qubits are engineered in systems that mimic the behavior of quantum particles, such as in superconducting circuits.
To function correctly, qubits must be kept at extremely cold temperatures. This is necessary to reduce noise and prevent errors that could result from unintended decoherence, where qubits lose their quantum properties and yield inaccurate results.
There are several types of qubits used in quantum computing today, each with its strengths and better suited to different tasks:
- Superconducting Qubits: These are created from superconducting materials that operate at ultra-low temperatures. They are known for their speed in performing computations and the precision with which they can be controlled.
- Trapped Ion Qubits: Trapped ion particles serve as qubits with long coherence times and high-fidelity measurements, making them reliable for complex operations.
- Quantum Dots: These small semiconductors capture a single electron to function as a qubit. They hold promise for scalability and are compatible with existing semiconductor technology.
- Photons: Photons, or individual light particles, are used to transmit quantum information over long distances through optical fiber cables. They play a crucial role in quantum communication and cryptography.
- Neutral Atoms: Neutral atoms, manipulated with lasers, are well-suited for scaling and performing operations in quantum computing.
To understand how qubits revolutionize problem-solving, consider this analogy: Imagine you are standing in the center of a complex maze. A classical computer, using traditional bits, would have to "brute force" its way out by trying every possible path one at a time, remembering which paths are dead ends.
Now, picture a quantum computer equipped with qubits. Instead of exploring each path individually, this computer can effectively take a bird’s-eye view of the entire maze, analyzing multiple paths simultaneously. It uses quantum interference, where the probability amplitudes of qubits overlap like waves, to cancel out incorrect solutions and highlight the correct path. This process is akin to eliminating all the wrong turns before ever setting foot in the maze, allowing the quantum computer to find the exit far more efficiently than its classical counterpart.
Quantum bits hold a superposition, enabling a quantum computer to approach problems in ways that classical computers simply cannot. This unique capability positions quantum computing to tackle problems that would otherwise be impossible to solve with traditional methods.
The Difference Between Quantum and Classical Computing
Classical computing, the backbone of our current technology, operates in a logical and sequential manner. This method is effective for a wide range of tasks, from everyday computing to advanced scientific calculations. However, classical computers, even the most powerful supercomputers, are inherently limited when it comes to tackling certain complex problems.
Quantum computing, on the other hand, operates on entirely different principles. By leveraging qubits and their ability to exist in multiple states simultaneously, quantum computers process data in parallel, using quantum logic and interference to explore vast datasets and solve problems at speeds that are orders of magnitude faster than classical computers for specific tasks.
The distinction between these two approaches becomes clear when considering how each handles complex problems. Classical computers are deterministic, meticulously working through every step of a calculation to arrive at a single, specific outcome. Quantum computers, however, are probabilistic, finding the most likely solution by exploring multiple possibilities at once. This difference enables quantum computers to address problems that would be infeasible for classical computers, such as modeling the behavior of atoms in a molecule or discovering new physics in a supercollider.
Despite their potential, quantum computers are not a replacement for classical computers in every scenario. For most tasks, traditional computing remains the most practical solution. In spite of that, for those exceedingly complex challenges—where countless variables interact in intricate ways—quantum computing offers a promising path forward. In fields like medicine, materials science, and finance, where understanding and predicting molecular behavior is crucial, quantum algorithms can simulate these behaviors more efficiently than classical supercomputers ever could.
As we stand and await the brink of a new era in computing, the future looks increasingly hybrid. Classical computers will continue to dominate in most applications, but the integration of cloud-connected quantum systems and hybrid ecosystems is already beginning to reshape industries. With major advancements on the horizon, quantum computing is set to unlock solutions to some of the world’s most challenging problems, potentially transforming entire sectors and paving the way for innovations we can only begin to imagine.
In the end, while classical computing will remain indispensable, quantum computing promises to take us beyond the limits of what we once thought possible, offering new tools to explore, understand, and shape the world in ways that could redefine the boundaries of human knowledge and capability imagine. What will be possible when its computing capabilities are bundled in a form-factor as easily portable as a Macbook?