Analyzing Our Galactic Anchors
Black holes are profound cosmic entities predicted by Albert Einstein's theory of general relativity. These are regions of space where gravity is so strong that nothing, not even light, can escape their grasp. The boundary surrounding a black hole is called the event horizon, and crossing it means entering an area where escape becomes impossible. While Einstein's theories suggested their existence, even he was somewhat skeptical. The concept of such gravitationally intense objects dates back to the 18th century, with John Michell's idea of "Dark Stars." It wasn't until the 20th century, with advancements in observational tools, that we found empirical evidence of black holes. Today, from stellar black holes to supermassive black holes at galactic centers, these enigmatic giants challenge our understanding of space, time, and the very fabric of the universe.
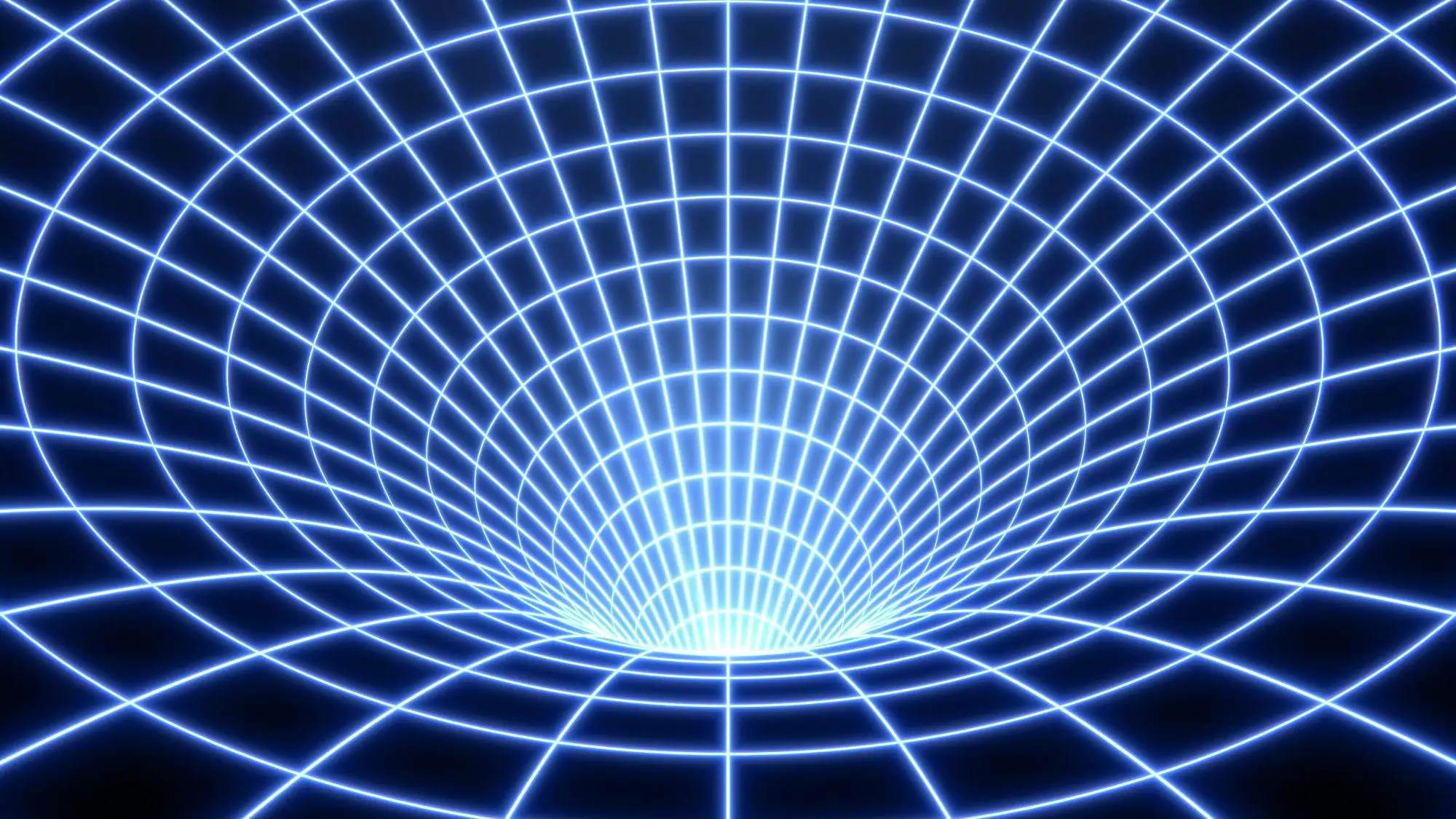
In Albert Einstein's general theory of relativity lies an audacious prediction: objects could become so massive and so dense that their gravitational pull becomes inescapable, even for light itself. Envision an entity so immensely powerful that it swallows light, the fastest thing in the universe, whole. To wrap our heads around such an idea, we must fathom an entity that not only bends space but also warps time to such an extent that all of time converges into a singular moment. This is the mysterious realm of the event horizon, the boundary of a black hole and once something crosses this boundary it can’t escape. This is where space, time, and light are curved to the extreme.
Let's try to make some sense of these cosmic entities.
Imagine wandering through a funhouse filled with distorted mirrors that warp your reflection, making you look tall, short, thin, or wide. Now, imagine a vast land with a similar funhouse effect, where familiar shapes and rules no longer apply. In this strange landscape, once-straight paths begin to twist and turn, and circles, which we learned in school are defined by the unchanging relationship between their circumference and diameter, no longer play by the rules.
This bewildering terrain hints at something more—a secret layer or "hyperspace", hyperspace is a theoretical higher dimensional space where the laws of our universe are different, but it's just beyond our grasp. While we might wish to leap into this hidden dimension to unravel its secrets, it remains an elusive mystery, visible only in the strange distortions it creates.
Lets dive a bit deeper into the idea of hyperspace. Imagine our universe as a vast sheet. Objects, like stars and planets, cause dimples in this sheet. Black holes, however, pinch it so sharply they seem to create a chasm. The question is: where does this chasm lead? Some theories propose a domain called hyperspace, a realm beyond our traditional three-dimensional space. This hyperspace might be a higher-dimensional space enveloping our universe.
When light is shone near a black hole, its trajectory bends due to the black hole's intense gravity. The Event Horizon Telescope captured this effect by photographing the shadow of a black hole. This shadow isn't the black hole itself, but a silhouette formed by light paths bending around it. Observing this shadow provides hints about the nature of hyperspace and the mysteries it might hold.
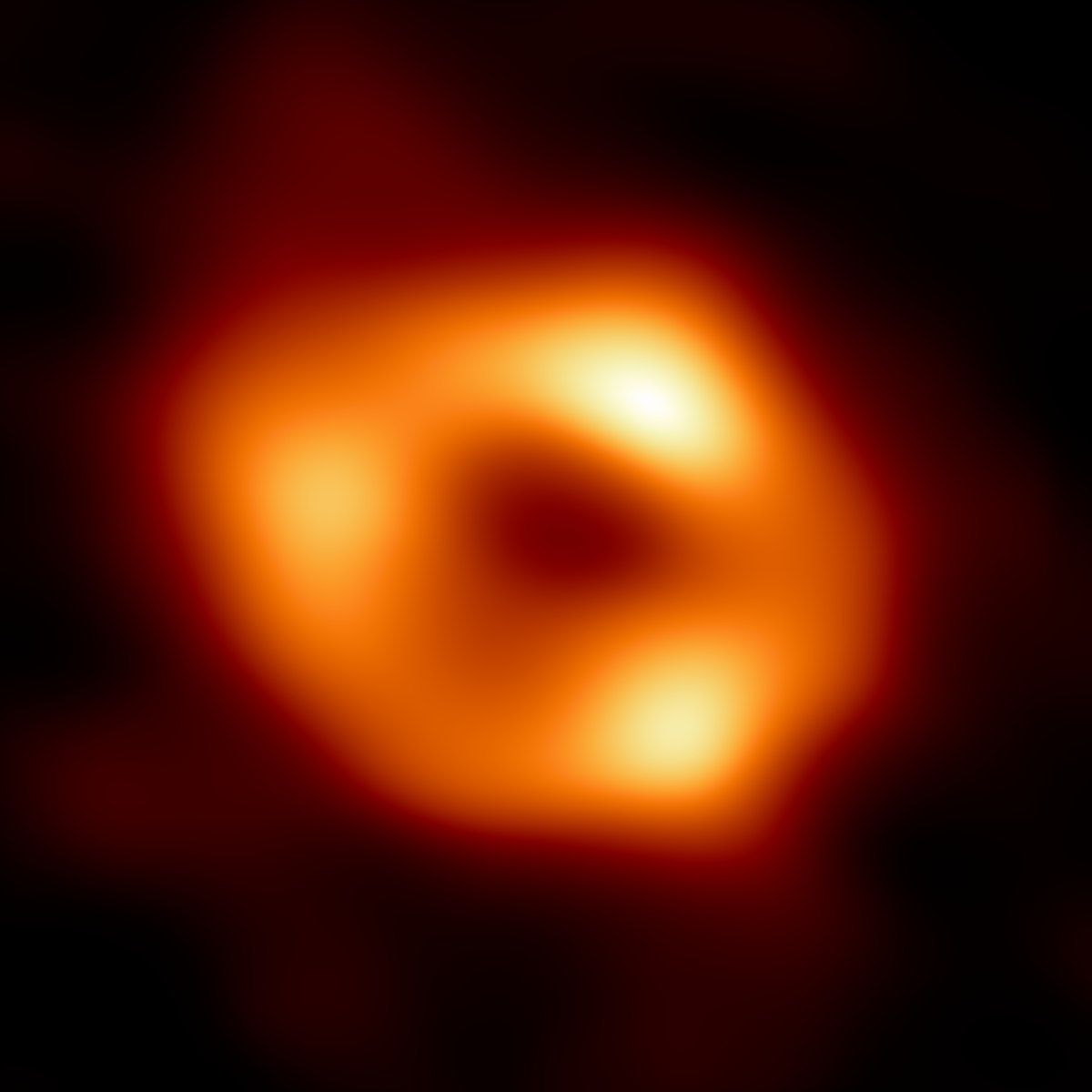
In essence, the black hole's shadow is a hint, much like ripples in water indicate a stone was thrown, even if we don't see the stone itself.
So when scientists study the puzzling regions that warp and bend around black holes, they encounter similar funhouse distortions in the very fabric of space itself. To understand these oddities, this is why they propose the existence of a higher-dimensional "hyperspace". Just like you'd stand puzzled in our warped funhouse landscape, scientists too grapple with understanding the mind-bending realms surrounding black holes.
Interestingly, while Einstein's groundbreaking theories foretold the existence of these cosmic singularities, he was, also paradoxically, among the skeptics. Long before Einstein's theory, in the 18th century, the idea of 'Dark Stars' had been speculated. John Mitchell, who coined the term after a thought experiment using Newtonian physics, pondered over a light particle being projected from the surface of a massive star. If the particle possessed sufficient velocity, it would eventually escape the gravitational grip of its star. However, if it couldn't reach escape velocity, it would be forever trapped.
Mitchell was no ordinary fellow. He trained at Cambridge University and engaged with intellectual giants of his age, like Benjamin Franklin, and was often deep in contemplation about the mysteries of the universe. Through his inquisitiveness, he envisioned stars of such intensity that they'd be invisible – the very epitome of darkness in a realm of light. However, the world wasn't quite ready for such a revelation, and Michell's brilliance was eclipsed by the luminaries of later ages.
First Evidence
The thought experiments by Mitchell and Einstein laid the conceptual groundwork with their revolutionary ideas, it was the advent of advanced observational tools in the latter half of the 20th century that would provide the tangible evidence, thrusting these celestial enigmas from theory into reality.
In 1964, the arrival of advanced x-ray satellites provided the first empirical evidence of a stellar black hole. In the constellation Cygnus, scientists detected an enigmatic object in a binary star system emitting a profusion of x-rays, yet conspicuously absent in visible light. Telescopic technology progressed, and by the 1970s, this object, dubbed Cygnus X-1 was observed again, revealing its true nature: a cosmic behemoth weighing in at 13 solar masses. This discovery heralded a new era of cosmic exploration, unearthing numerous active black holes peppered throughout the universe, including the gargantuan supermassive black holes reigning at the heart of galaxies.
How are they created?
Stellar black holes, like Cygnus X-1, are the final stages of massive stars that have succumbed to their own gravitational forces and collapsed. Observations suggest that Cygnus X-1 likely originated from a star that underwent a near-total collapse. A looming giant in our night sky, Betelgeuse in the Orion constellation, possesses a substantial mass of about 16.5 solar masses. As it cools and struggles under its immense weight, this red supergiant offers us a front-row seat to the tumultuous life cycle of massive stars nearing their end.
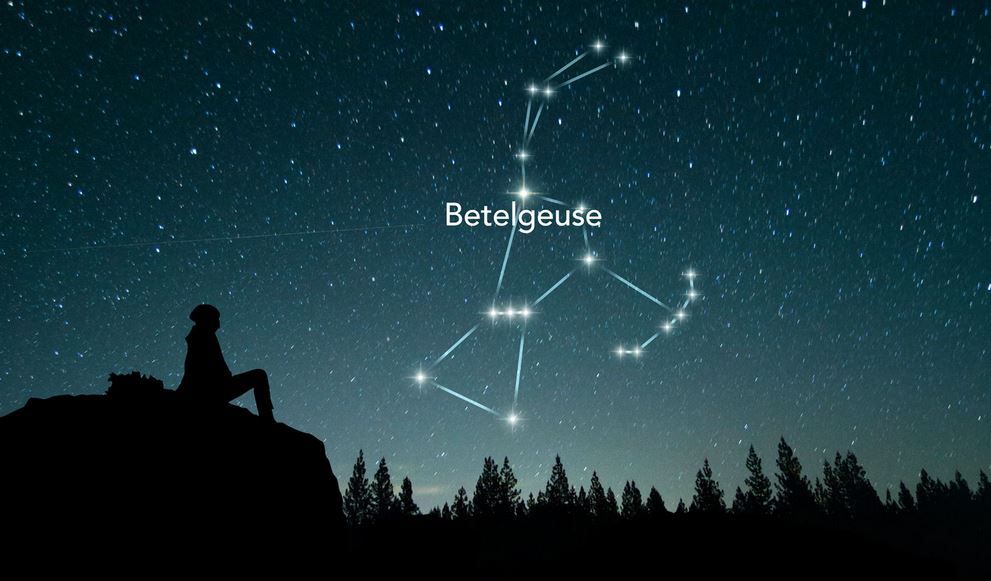
Moving beyond individual stars and into the grand scale of galactic centers, we encounter phenomena that were once deeply puzzling. Central to many galaxies are what we now identify as quasars or active galactic nuclei — radiant rings of light. Their nature remained a mystery until insights from the early 1970s began to shed light on these luminous heartbeats of galaxies.
Sagittarius A*
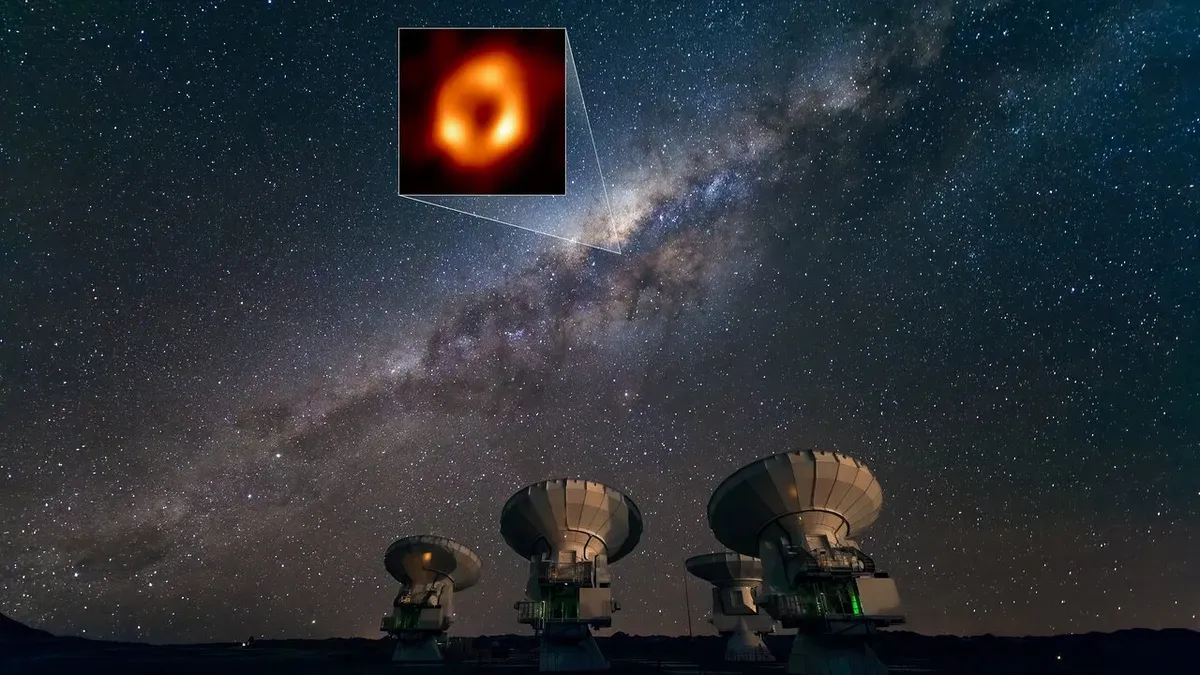
Back in 1974, in Green Bank, West Virginia, astronomers Bruce Balick and Robert L. Brown made a groundbreaking discovery using a radio interferometer on the nearest quasar, A radio interferometer merges signals from several antennas to better study radio sources in space. They detected powerful radio emissions emanating from the heart of the Milky Way galaxy, pointing directly to a compact, source that challenged conventional astronomical wisdom. The emissions acted as a "smoking gun," alluding to a disk of material orbiting around a colossal, unseen entity at near lightspeed, and thus began a passionate quest to understand this cosmic enigma. This pivotal observation unveiled the existence of Sagittarius A*, marking our first tangible evidence for a supermassive black hole.
Sagittarius A* is estimated to be a few million times the mass of the Sun. Mostly dormant, its considerable size challenges our traditional models of how these black holes are birthed and grow. While one might be tempted to think such a behemoth evolved from a collapsing star, this seems improbable. Lending further complexity, the James Webb Space Telescope (JWST) has unveiled fully formed spiral galaxies, complete with distinct galactic arms and quasars at their centers, from a time just 3 billion years after the Big Bang. This discovery raises questions: How did such massive black holes form so early in the universe’s history?
To better understand, consider this analogy: If a stellar black hole is akin to a collapsed mansion – imposing, intriguing, and a standout in its vicinity – then a supermassive black hole would resemble an entire flattened city, dwarfing the mansion with its vastness. A stellar black hole like Cygnus X-1 might boast up to 21 times the mass of our Sun. In contrast, supermassive black holes, such as the one anchoring our Milky Way, can be millions to billions times more massive.
In the cosmic theater, stars like Betelgeuse, impressive as they might be, are but supporting actors. They may eventually evolve into stellar black holes, but they pale in comparison to the colossal entities residing in galactic centers. The universe, with its vastness and enigma, persistently reminds us of our limited understanding.
So, the question looms: How did these black holes achieve such immense proportions? Do they have an insatiable appetite? The growth and nature of these cosmic giants remain an intricate astrophysical puzzle, with scientists often grappling to reconcile their mass and size with existing theories.
The final parsec problem
Most of these Islands of billions of stars out in the universe harbor supermassive black holes. So, when two galaxies merge, like the Andromeda and Milky Way merge in a 4 billion years, its assumed that their supermassive black holes will also merge right? The math says no. They will possibly encounter a resistance known to astronomers as the final parsec problem. The 'final parsec problem' is an intriguing puzzle where two supermassive black holes come close to merging but get stuck about 3 light years apart.
Because they may be restrained by the laws of dynamical friction. Dynamical friction is like cosmic ‘drag’, when massive objects move through space, they create a sort of gravitational wake, slowing them down. In astrophysical contexts, dynamical friction refers to the transfer of energy between massive objects and their surrounding field of smaller objects due to gravitational interactions. We launched spacecraft like Voyager 1 & 2 from Earth's orbit using this gravitational assist technique, the spacecraft effectively "borrows" energy from Earth to boost its speed. In this process, although the Earth loses a minuscule amount of its kinetic energy - the energy an object has because of its motion, the spacecraft gains a significant boost due to the Earth's immense gravitational pull. The vast difference in mass between the Earth and the spacecraft makes this energy transfer efficient. As a result, the Voyager crafts have been propelled to the outer reaches of our solar system, demonstrating the profound effect of this energy exchange.
Similarly, When massive objects, like supermassive black holes (SMBHs), move through a field of smaller objects, especially after two galaxies merge, they tug on surrounding stars. This tug gives stars a boost of energy, causing the stars to shoot away, while the black holes lose energy and drift closer together. This drifting helps two black holes get really close, setting the stage for a potential cosmic dance where they might merge.
However, here's the twist: as they get super close, they run out of enough nearby stars to interact with, so they can't lose enough energy to complete their merger. They get stuck about a parsec apart — and to clarify, a parsec is a unit of distance in space equal to about 3.26 light years — creating what's known as the "final parsec problem". It's like two dancers wanting to come together, but something mysterious keeps them just out of arm's reach.
So if they don't merge, what can be an explanation?
Black holes, especially supermassive ones, can be likened to cosmic vacuums, exerting a powerful gravitational pull on anything that ventures too close. This immense gravitational force not only attracts matter but can also accelerate nearby gas and dust to high velocities, making them emit x-rays and other forms of radiation. As these materials spiral towards the black hole, they form an 'accretion disk'—a swirling ring of matter that heats up due to friction. This process can cause the disk to shine intensely, often making it one of the most luminous objects in its host galaxy.
It's crucial to differentiate this activity from the black hole itself 'devouring' matter. While a black hole's immense gravity draws matter towards it, not everything that gets close ends up inside the black hole. Instead, a lot of the material circles around it in the accretion disk. The heat and radiation generated from this rapidly swirling matter can push other materials away, acting as a cosmic deflector shield, preventing everything from being sucked in immediately. This dynamic interplay between attraction and radiation pressure can create a wide array of fascinating phenomena around black holes.
Messier 87: a cosmic behemoth
Within the Virgo supercluster, home to us and approximately 2,000 other galaxies, the galaxy Messier 87 (M87) houses a black hole of immense proportions, weighing over 6 billion times our Sun’s mass. In comparison, our galaxy's heart, Sagittarius A* (Sgr A*), has a mass of 'only' 4 million times that of the Sun. The Virgo cluster houses M87 has long been an astronomical point of interest due to its significant influence over its immediate and distant galactic neighbors, including our Local group of galaxies containing the Milky Way.
The SMBH in M87 is believed to be considerably more active, evidenced by phenomena like the powerful jets of particles shooting out from its vicinity. Herber Curtis, in 1918, first observed M87 and made note of these prominent streams of gas and dust emanating from its black hole, spanning several light years. Such features indicate the dynamic processes occurring around the black hole, contributing to its overall activity. Again, It's important to understand that this activity is not strictly the same as the black hole's accretion rate, which measures how quickly matter is being pulled into the black hole. While increased activity can sometimes be a result of high accretion, a black hole can also be highly active due to other surrounding processes, even with a modest rate of matter intake. Therefore, interpreting M87's vigorous jet streams and luminosity, it's essential to differentiate between the general activity and the specific rate at which it's accumulating matter.
A supermassive black hole's size is unlikely a result of its accretion rate alone.
Ellipticals, Spirals, and the mystery of Andromeda
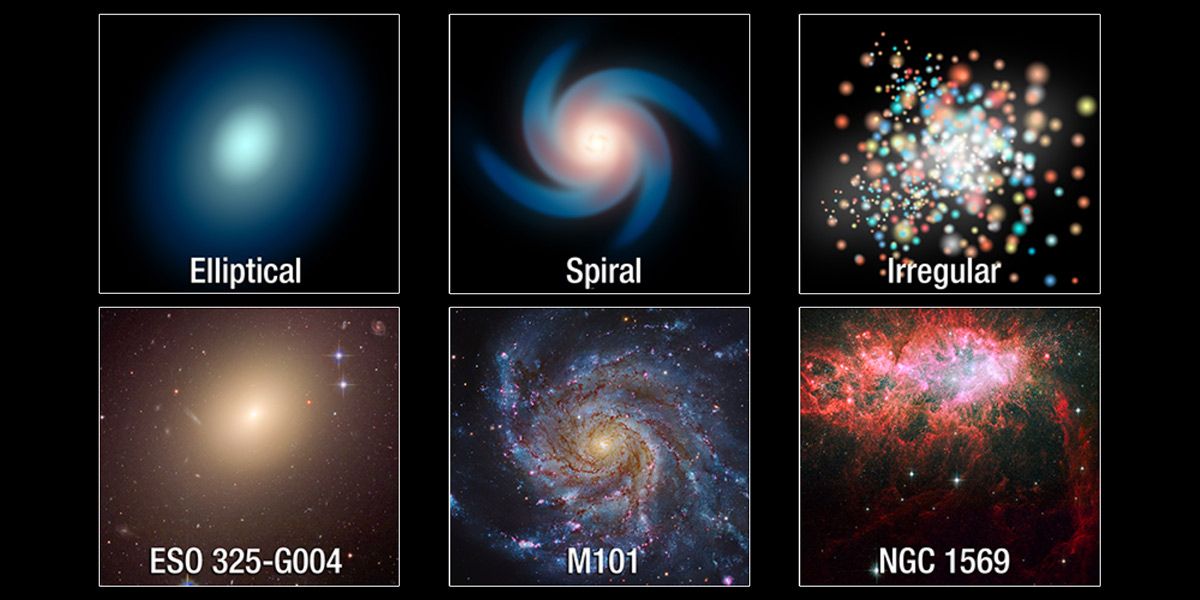
While the majority of galaxies, like our Milky Way, are spirals, M87 is an elliptical galaxy. These ellipticals, believed to be the products of galactic mergers, are typically larger and house heftier black holes. But as any attentive spectator of the cosmos would affirm, the plot thickens with twists. Enter stage: the Andromeda galaxy, not too distant at 2.537 million light-years. While it mirrors the Milky Way's spiraled elegance and size, at its heart beats a different rhythm—a black hole of an astonishing 100 million solar masses, guarded not by the exuberant stars of youth but by the wise, red ancients, narrators of a gentler past. This narrative of M87 and Andromeda serves as a poignant reminder that, akin to our own history, the cosmos spins stories rich in mystery, drama, and revelations.
The Ultra Massive Enigma
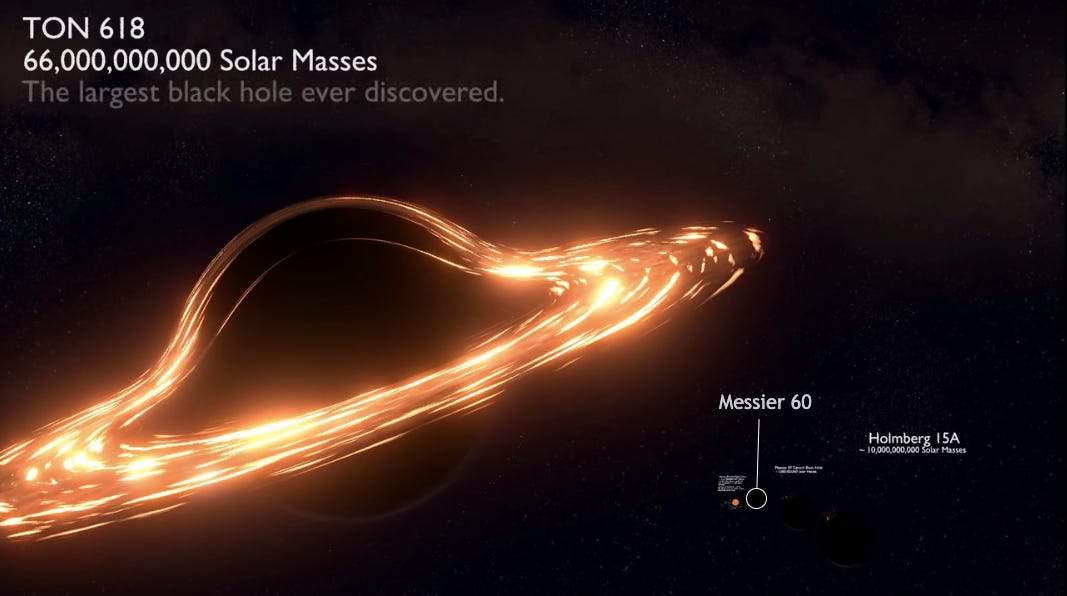
Venturing further into the cosmic realm, we encounter even greater mysteries. Ultra Massive Black Holes (UMBHs), like the one at the heart of TON 618 located a staggering 10 billion light years away, defy our understanding. Tipping the scales at 66 billion solar masses, its very existence raises fundamental questions about the universe's evolution.
As we push the boundaries of our cosmic understanding, one thing becomes evident: the universe, with its supermassive and ultra massive black holes, remains an enigma. Our journey to unravel these celestial mysteries has only just begun, and with every discovery, newer questions arise, prompting us to delve deeper into the cosmic unknown.